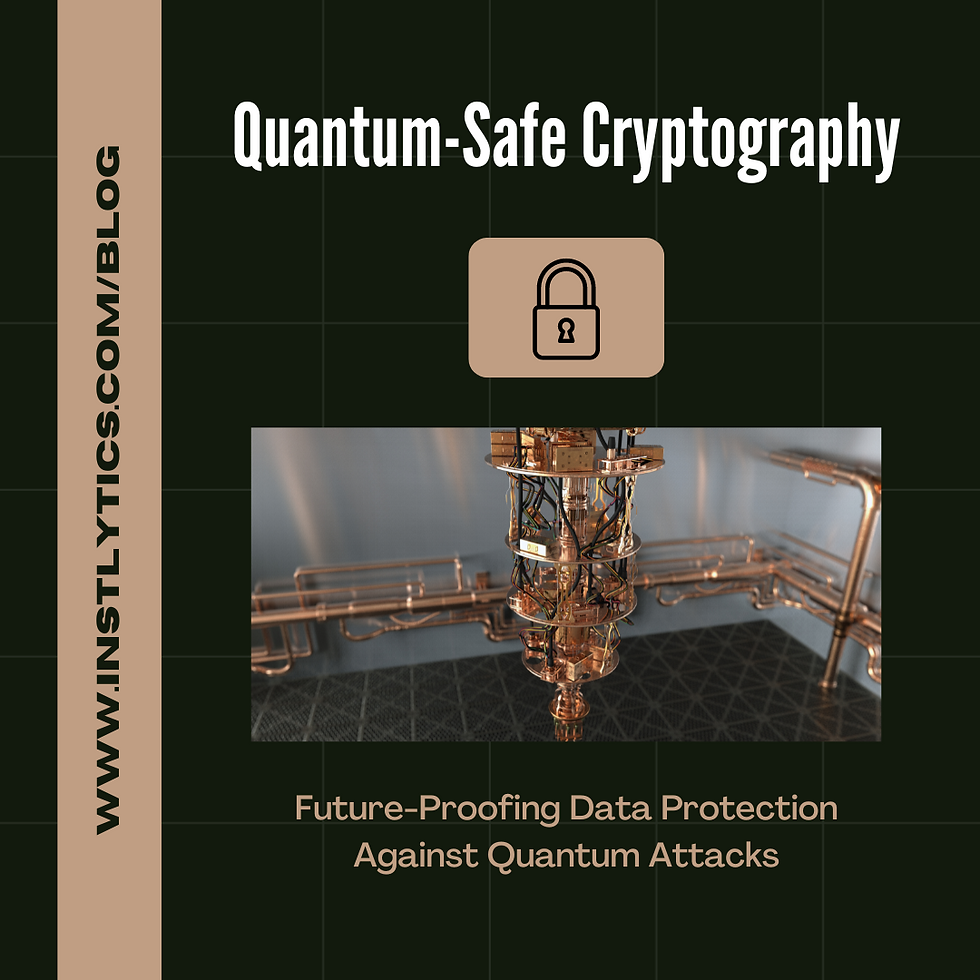
In the rapidly evolving landscape of cybersecurity, the emergence of quantum computing poses unprecedented challenges to conventional encryption methods. While traditional cryptographic algorithms provide robust protection against classical computing threats, they are vulnerable to attacks from quantum computers, which have the potential to break current encryption schemes with exponential speed and efficiency. Quantum-safe cryptography, also known as post-quantum cryptography, offers a viable solution to this looming threat by developing encryption algorithms that are resistant to quantum attacks. In this article, we delve into the significance of quantum-safe cryptography, its underlying principles, and its role in future-proofing data protection against quantum threats.
Understanding the Quantum Threat:
Quantum computing harnesses the principles of quantum mechanics to perform calculations at speeds exponentially faster than classical computers. While quantum computing promises revolutionary advancements in fields such as optimization, simulation, and machine learning, it also poses a significant threat to traditional cryptographic systems. Quantum computers have the potential to solve complex mathematical problems, such as integer factorization and discrete logarithms, which form the basis of widely used encryption algorithms, including RSA (Responsive Search Ads) and ECC (Elliptic Curve Cryptography). As a result, quantum computers could render existing encryption schemes obsolete, compromising the security of sensitive data and communications.
The Need for Quantum-Safe Cryptography:
Given the imminent threat posed by quantum computing, there is an urgent need to develop encryption algorithms that can withstand quantum attacks. Quantum-safe cryptography aims to address this challenge by designing cryptographic primitives and protocols that remain secure against both classical and quantum adversaries. By transitioning to quantum-safe encryption algorithms, organizations can future-proof their data protection strategies and mitigate the risks associated with quantum computing advancements.
Principles of Quantum-Safe Cryptography:
Quantum-safe cryptography relies on several fundamental principles and approaches to ensure security in the presence of quantum adversaries:
Mathematical Hardness: Quantum-safe encryption algorithms are based on mathematical problems that are believed to be computationally hard, even for quantum computers. These problems, such as lattice-based cryptography, code-based cryptography, and hash-based cryptography, offer a foundation for building encryption schemes that resist attacks from both classical and quantum adversaries.
Quantum-Resistant Algorithms: Quantum-safe encryption algorithms are specifically designed to withstand attacks from quantum computers. Unlike traditional cryptographic algorithms, which rely on the difficulty of factoring large integers or solving discrete logarithm problems, quantum-resistant algorithms leverage alternative mathematical structures and computational assumptions that are believed to be secure against quantum attacks.
Post-Quantum Standards: Recognizing the importance of transitioning to quantum-safe cryptography, standards bodies, and organizations, such as NIST (National Institute of Standards and Technology), are actively evaluating and standardizing post-quantum encryption algorithms. These efforts aim to establish a set of quantum-resistant algorithms that can serve as replacements for existing cryptographic standards, ensuring interoperability, compatibility, and adoption across different systems and applications.
Applications of Quantum-Safe Cryptography:
Quantum-safe cryptography has broad applications across various domains, including:
Secure Communication: Quantum-safe encryption algorithms can be deployed to secure communication channels, such as email, messaging platforms, and virtual private networks (VPNs), against quantum eavesdropping and interception attacks. By encrypting data using quantum-resistant algorithms, organizations can protect sensitive information and ensure confidentiality and integrity in communication networks.
Data Protection: Quantum-safe cryptography enables organizations to protect sensitive data, such as financial records, intellectual property, and personal information, from quantum threats. By encrypting data-at-rest and data-in-transit using quantum-resistant encryption algorithms, organizations can safeguard their assets and comply with regulatory requirements for data protection and privacy.
Digital Signatures: Quantum-safe digital signature algorithms allow entities to authenticate and verify the integrity of digital documents, transactions, and software updates in a quantum-resistant manner. By generating digital signatures using post-quantum cryptographic schemes, organizations can ensure non-repudiation, tamper-proofing, and trustworthiness in digital interactions and transactions.
Challenges and Considerations:
While quantum-safe cryptography offers promising solutions to mitigate the risks posed by quantum computing, several challenges and considerations must be addressed:
Performance and Efficiency: Quantum-safe encryption algorithms may have different performance characteristics and resource requirements compared to traditional cryptographic algorithms. Organizations must evaluate the performance, efficiency, and scalability of quantum-safe encryption schemes to ensure compatibility with existing systems and applications.
Interoperability and Adoption: Transitioning to quantum-safe cryptography requires coordination and collaboration among stakeholders, including standards bodies, vendors, developers, and end-users. Organizations must navigate the challenges of interoperability, migration, and adoption to integrate quantum-safe encryption solutions seamlessly into their existing infrastructure and workflows.
Quantum-Safe Key Management: Quantum-safe encryption algorithms require robust key management practices to protect cryptographic keys from quantum attacks. Organizations must implement secure key generation, distribution, storage, and rotation mechanisms to safeguard sensitive keys and ensure the long-term security of encrypted data.
Conclusion:
In conclusion, quantum-safe cryptography is essential for future-proofing data protection against the impending threat of quantum computing. By developing encryption algorithms that are resistant to quantum attacks, organizations can safeguard sensitive data, secure communication channels, and ensure the integrity of digital transactions in an increasingly quantum-enabled world. As quantum-safe encryption standards continue to mature and gain adoption, organizations must proactively assess their cryptographic infrastructure, implement quantum-safe encryption solutions, and stay vigilant against emerging threats posed by quantum adversaries. Through strategic investments in quantum-safe cryptography, organizations can mitigate cyber risks, preserve trust in digital systems, and uphold the confidentiality, integrity, and availability of their data assets in the face of quantum computing advancements.
Comments